Case
Study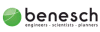
Red Gate Pedestrian Bridge
-
Novel cable
stayed pedestrian/cycle bridge suspended beneath road bridge
-
Detailed global
modelling of bridge and local modeling of cable connection
assembly
-
Pedestrian
footfall analysis to ascertain maximum
acceleration values for comparison with pedestrian comfort
levels.
enesch provided Phase II engineering and Phase III construction
management services for the project. - See more at:
http://web.benesch.com/red-gate-road-bridge-named-public-works-project-of-the-year-pages-171.php#sthash.TosSj4Nq.dpuf
US consultant, Benesch, used LUSAS Bridge analysis software to
assist with its design of a novel cable stayed pedestrian bridge that
is suspended
beneath the Red Gate Road Bridge that crosses the Fox River in the northern
part of St Charles, Illinois. Load take-off and sensitivity
analyses were carried out which, along with a staged erection analysis,
helped to derive main beam camber values. Extreme loading events were
assessed, and eigenvalue analysis and subsequent pedestrian footfall
analysis indicated the structure could be susceptible to pedestrian
induced vibration,
resulting in the eventual installation of a tuned mass damper system.
Overview
Red Gate
Bridge connects IL Route 31 and IL Route 25 across the Fox River
in the northern part of St Charles - a western suburb of Chicago,
Illinois. The two-lane road
bridge, completed and opened to public in late 2012, supports an innovative
cable-stayed pedestrian bridge from, and inside its concrete piers.
The footbridge, which commenced construction once the piers has been
built, but was completed later, was officially opened for public use
in June 2013. It separates cyclists and pedestrians from the vehicular
traffic and provides access to various forest preserves, park
facilities and regional trails in the area.
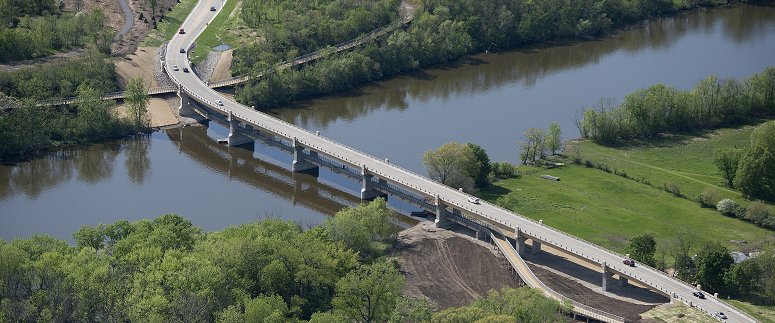
The
footbridge consists of four spans
of 150-0 (45.7m) and two anchor spans of 66-3 (20.2m)
each. The western anchor span and the adjacent span are curved with a
radius of 575 (175m). The steel
superstructure consists of two main W24x104 (610mm deep x 324mm wide x
157kg/m) longitudinal stiffening 'I' beams, spaced 14-6 (4.4m) apart, and W12x30
(315mm deep x 165mm wide x 45kg/m) transverse floor 'I' beams spaced at 7-6 (1.47m) that are laterally braced. The
12-10 (3.9m) wide deck is a 7 (178mm) thick
non-composite-acting concrete slab, cast on stay-in-place corrugated metal decking,
that spans between floor beams. Three cables, each
attached to an upper and lower anchorage connection, support the ends
of each longitudinal beam. Transverse beams at the cable connection
locations are W24x62 (603mm deep x 178mm wide x 94kg/m) 'I' beams in order to resist the torsion created by the
lateral inclination of the supporting cables. Cable anchorages at the
piers are outside the plane of the main beams and the cables are
laterally inclined by 8.5 degrees to the vertical plane. The piers
themselves are skewed seven degrees to align with the flow of the
river, meaning that careful detailing and positioning of the upper and
lower anchorage plates was required.
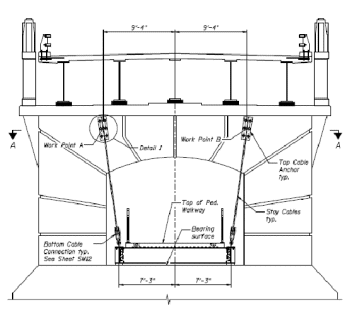
Schematic
section at pier. |
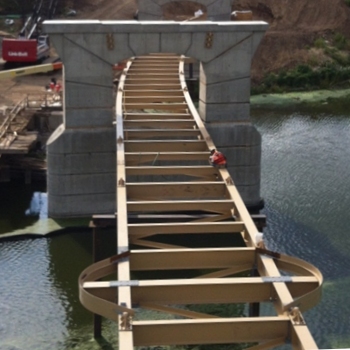
Steel
deck framing was erected prior to installation
of road bridge beams |
Modelling
in LUSAS
LUSAS was used to assess the behaviour of the structure to meet the criteria of the 5th Edition 2010 AASHTO Bridge Design Specifications along with the 2009 AASHTO Guide Specifications for the design of pedestrian bridges. Analyses included self-weight, pedestrian live load, H10 vehicle loading, wind load, and unbalanced live loading due to extreme events such as eccentric pedestrian loading arrangements and cable breakages. A model of the complete footbridge was built using thick beam elements to represent the structural steel framing, bar elements for the cable stays, and thick shell elements to model the concrete deck. Support conditions varied to match those of the structure with abutments modelled with only a vertical reaction, piers 1, 3, and 5 having expansion bearings allowing longitudinal movement, and piers 2 and 4 using fixed bearings that allowed longitudinal movement. Once created, a load take-off was carried out in LUSAS to ascertain the maximum factored cable axial force, as well as the maximum service load. LRFD analysis and allowable strength design checks were both performed, so that the cables would meet both requirements. Static forces recommended by a wind study were included in the analysis model to incorporate the effects of wind on the structure. Modelling of the full erection sequence produced deck displacements that determined the 2-1/8" (54mm) pre-cambering value for the main beams.
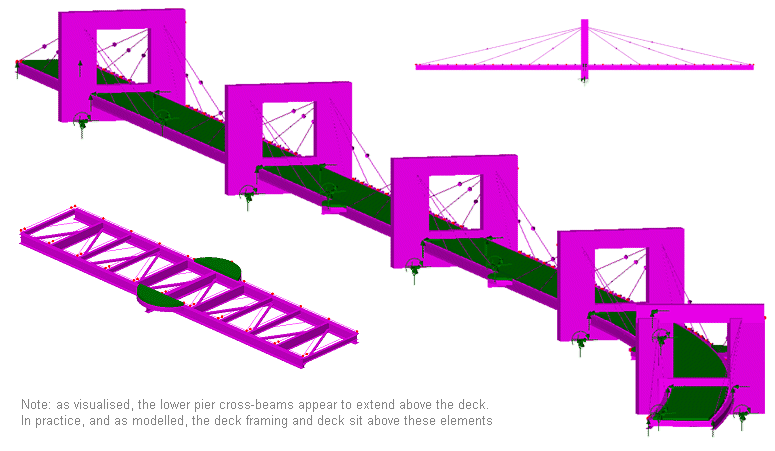
|
LUSAS model
showing cable arrangement at each pier and typical steelwork
framing arrangement |
Upper and lower
anchorage connections
Upper and lower anchorage connections are steel plated assemblies of various section thicknesses. For the upper connection the main objective was to avoid transmitting any tensile forces to the concrete pier so, to achieve this, six debonded 1
(25mm) diameter high-strength bars pass through the pier to the anchorage assembly on the other side to transfer the load as a compressive force on the anchorage plate on the adjacent side of the pier. Shear force is transferred to the pier via shear studs welded to the back of the mounting plate. A LUSAS model of an upper anchorage helped to verify the stresses and the forces in the debonded bars. The lower connection of the cables to the main girders uses an open bridge socket connected to an anchorage assembly bolted to the top flange of the girder.
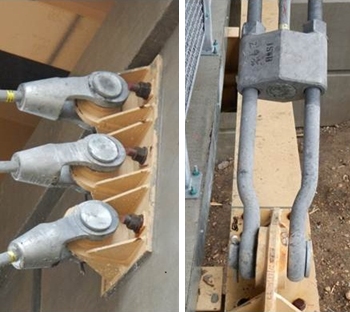
|
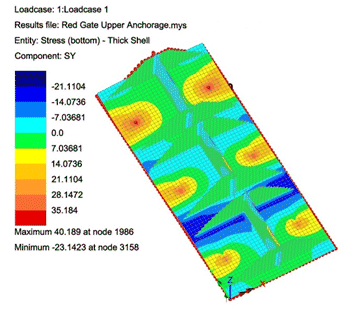
|
Upper and lower
cable connection details |
LUSAS model of
upper cable connection assembly |
Sensitivity
analyses and extreme loading assessments
A
number of sensitivity analyses were performed with LUSAS to better
understand the structure and the relative effect that certain
variables had on the overall behaviour. These
included looking at the connection fixity of the transverse floor
beams; investigating the effect of modelling the true
eccentricity of
the girders relative to the deck and cable connection point
on the overall behaviour of the model; and analysing the effect on the
cables from using different vertical and horizontal modelling
locations
for the cable connections. For the latter, it
was determined that modelling the pier connections as 3 separate
points and modelling the correct horizontal distance between the cable
anchorages, rather than joining them direct to each thick beam element
representing the pier, was both more realistic, and provided more accurate
results.
In
addition to strength, serviceability and sensitivity checks,
extreme loading events such as eccentric pedestrian loading
and loss of cables were also assessed.
|
Real position
modelling of cable connection locations |
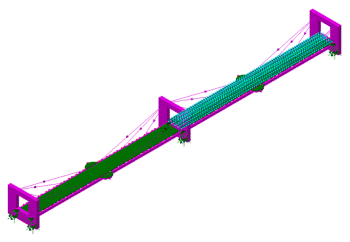
|
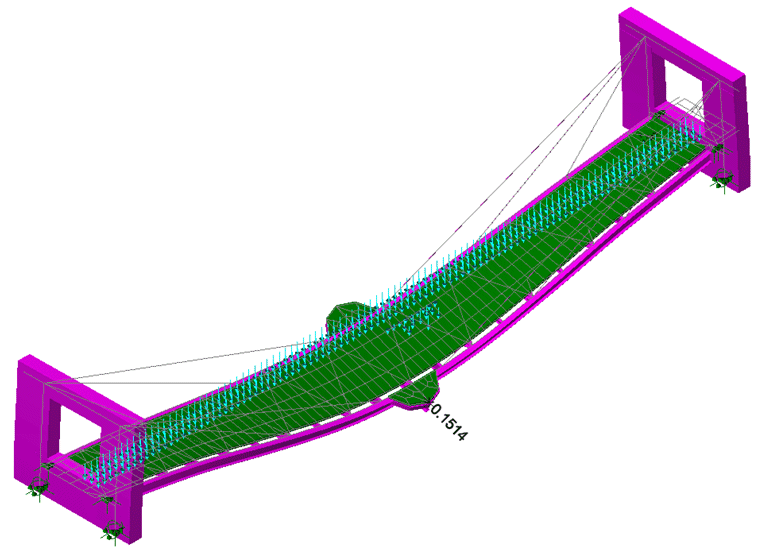
|
One span loaded with pedestrians with adjacent span empty. |
Eccentric
pedestrian loading on one side of
the deck. |
Pedestrian
footfall analysis
An eigenvalue analysis derived the
key fundamental mode shapes and frequencies, and
showed the first mode with a significant vertical mass
participation had a frequency of 2.11 Hz.
A dynamic analysis modelled the behaviour
of the structure to various moving and pulsating pedestrian loads.
Hossam Abdou, Vice President of Alfred Benesch and Company said:
"Although the AASHTO Guide for Design of Pedestrian Bridges
addresses the issue of vibration it
appears to be geared more toward stiffer girder structures rather than
slender and flexible cable structures. The Guide uses formulas that relate the weight of the structure to its natural frequency, however,
it fails to address the size of the pedestrian traffic as it relates to the vibration induced and whether the resulting acceleration is within
permitted limits for the traffic." Because of this the model was
converted into SI units, appropriate
Rayleigh damping parameters were specified to represent
the damping behaviour of the materials / structure, and the LUSAS
pedestrian load wizard was used to apply vertical oscillating
pedestrian loading to the model in accordance with BS EN 1991-2 2003.
This automatically created the numerous loadcases required to model the passage of the pedestrian groups across the
structure.
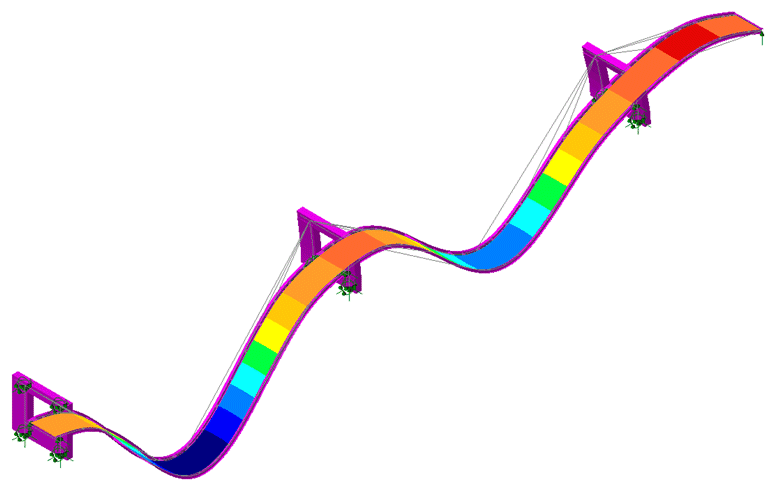
|
Eigenmode
used for footfall analysis |
A
plot of pedestrian load versus time and pedestrian load versus
distance was automatically created by LUSAS, showing the sinusoidal
load curve for the dynamic analysis. From
this, a maximum vertical acceleration of 0.1728 m/s2
(1.76% g), and a maximum resultant acceleration of 0.1735 m/s2
were seen. The LUSAS analysis indicated that the maximum
pedestrian level guidelines could be met but, as a
precautionary measure, the deck framing had been laid out such that
tuned mass dampers could be accommodated if they had been required.
Subsequent field testing involving placing people on the completed structure indicated that
uncomfortable vibrations might indeed be experienced and, as a result, the City of St.
Charles
ultimately decided to proceed with the installation of a damper
system to increase pedestrian comfort.
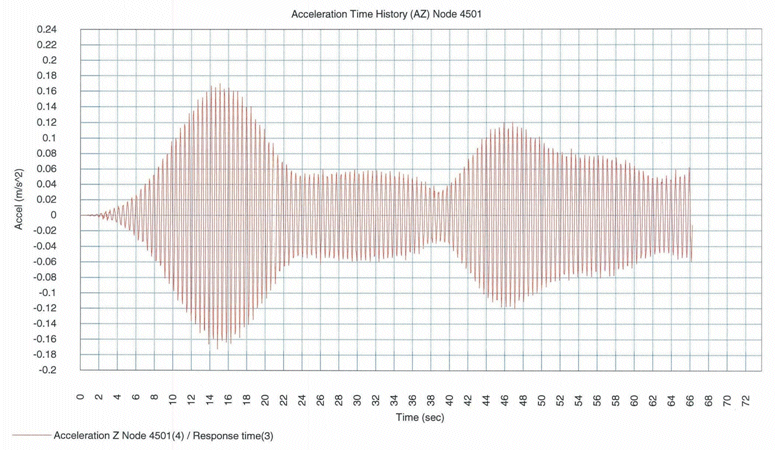
|
Maximium
acceleration time history for controlling node |
"Using LUSAS
allowed us to accurately capture the behaviour of a very complex
structure that included a curved and skewed alignment with cables
that had both vertical and lateral inclinations." Hossam
Abdou, Vice President, Alfred Benesch and Company.
Find out more
Other LUSAS Bridge case
studies:
|
|
Software Information
|